SUBHEAD: Is it possible that technology as simple as an Easy Bake Oven can reverse climate change?
By Albert Bates on 2 April 2017 for The Great Change -
(http://peaksurfer.blogspot.co.uk/2017/04/the-cool-lab.html)
Image above: Illustration of "biorefinery" concept that can be applied to utilization of bamboo forestry. From original article.
[IB Publisher's note: The "Cool Lab" technology that Albert Bates describes in this article includes ideas from holistic thinking, permaculture, and technologies like biochar that could transform agriculture and our use of technology, and be the solution to Global Warming, Climate Change and our own extinction. Get on board!]
In Permaculture the first stage of any design is protracted observation. What does a biological system have in over-abundance? What is scarce? How will it restore balance? What are the obstacles?
Let us say that an impoverished village in Haiti risks being carried away by mudslides that follow brush fires where the forest has been cut down to supply wood for shelter and cooking.
What things are scarce? In no particular order:
The hillside needs to be planted with vegetation. It is especially important that the hilltops be forested.
Image above:Principles and techniques Refenerative Agriculture to improve whole agroecosystems. From original article.
A keyline analysis will show us where water wants to go when it rains, and how best it can be held high in the landscape and directed both to subsurface flows and to dam storage for uses in the dry season. Alley cropping along the contours follows hand-cut swales (or machine cut where financial capital substitutes for social capital).
The berms are planted with successional understory (in this tropical example, pineapple, cassava, ginger, allspice, coffee and medicinal herbs), mid-level canopy banana, papaya, moringa, cacao, mulberry, tree legumes of mimosa, cassia, and pea subfamilies, chaya, climbing vines such as vanilla, dioscorea, cucumber, chocho and pasaflora, and eventual overstory of coconut, jackfruit, breadfruit, breadnut, ramon, samwood, mahogany, cedar, bamboo, peach palm, etc.
Between the alleys are seeded perennials such as callalu, okra, sorghum, and supergrasses like kernza (Thinopyrum intermedium), sunn hemp (Crotalaria juncea), pennisitum and pearl millet hybrids (Tembo), brassica napus, amaranth, etc., as well as familiar food crops such as maize, rice, yam and beans, where soils and water supply are well suited.
As much as possible, the planting process can be accompanied by biofertilizers having a high percentage of finely pulverized biochar, activated indigenous microorganisms, some immediate food for those microbes (such as composted food wastes and manures), and minerals keyed to redress local soil deficiencies.
If these biofertilizers are not immediately available for the first plantings, they can always be added later, as a byproduct of the early harvests.
Water in storage on the hillsides is edge-planted with Acoris, a plant that inoculates the water with a mosquito-larvae destroying resin. As the Acoris matures, pools and dams progress from being mosquito generating to mosquito decimating.
In the lowlands, water that overflows from catchments above is directed into chinampas, constructed wetlands composed of alternating islands and channels and rotating between aerobic (horizontal and vertical flow reedbeds) and anaerobic (settling lagoons) seeded with aquatic and semi-aquatic plants (taro, Chinese water spinach, lotus, azola, wild rice) and freshwater fish (aquaculture).
Acoris for mosquito control can also be planted here, but the fish do most of that work already, so the plant is only needed in mudflats and places fish cannot go.
The appearance of this microbiome also augurs the reappearance of frogs, peepers, lizards, dragonflies, water birds, bats, turtles, and forest mammals who venture to the water’s edge to drink.
Within the first season, the hillside mud problem is erased, deforestation is reversed, and food scarcity begins to be alleviated from the fast-yielding varieties of annuals, perennials and fish.
Productive employment can expand this system as much as available land permits, even on relatively steep hillsides. Resentment diminishes, and with it, superstition.
Within the village a regenerative, biological energy system arrives to replace the fossil fuel (diesel electric) grid-based source that previously had supplied electricity only intermittently, occasionally dimming lights and frying phone chargers and boom boxes.
This system consists of a biomass furnace, running on the woody wastes from coppice (the moringa, jackfruit and cassava plantation), coconut, rice or other shell crops, pelletized supergrasses and other biomass after food harvest or extraction of leaf protein, vitamins and useful fiber.
The loading dock at the biorefinery receives raw materials second-harvested from the farms.
Leaves of tropical legumes (Leucaena Zeucocephala, Vigna unguiculata, Clitoria ternatea, Desmodium distortum, Psophocarpus tetragonolobus, Macroptilium lathyroides, Phaseolus calcaratus, Brassica napus, and Manihot esculenta, for instance) are taken by conveyor and chopped into 2-cm pieces, soaked in 2-percent sodium metabisulfite, disintegrated in a hammer mill and pressed in a single-screw press.
The expressed juice is heated with steam (produced by the furnace) and protein coagulum collected, centrifuged, and pressed, then spread in a thin layer on glass plates and dried in an air-filtered, dehumidified room. It is then collected as a powder and containerized to be used or sold as a feed supplement.
At its most basic level, high-protein, high-quality leaf protein fractionation is simple. Production is geared to consumption by farm animals to remove some of the food safety, preservation and storage concerns.
Later improvements can produce dried leaf extracts for human consumption but higher capital costs are incurred and clean-room protocols by workers become essential.
Following leaf-protein extraction, the dried mash from the press is used as a feedstock for the furnace, where it joins other dried agricultural wastes: coppice wood, prunings, bamboo thinnings, pallets, cardboard boxes, coconut coir, nut and rice husks, etc.
All of this is pyrolyzed, the heat captured to run both the leaf protein process and produce electricity, and co-products (fractionated volatile gases, wood vinegar) drawn off before the final product — high quality biochar — remains.
The biochar is quenched (preferably with urine because that adds a 30% fertility gain), pulverized, and charged (blended with microbe-rich aerobic compost) to make a potent “cool” biofertilizer.
Alternatively, it is kept at food-grade and sold as a dry product for use as a food supplement, animal feed probiotic, water filtration medium or deodorizer. At less-than-food-grade it can be used as a litter amendment to reduce smells in animal enclosures, improve the fermentation of silage, or go into a variety of natural building materials — paints, dyes, plasters, wallboard and bricks.
And it can always become biofertilizer, even after undergoing one or more of these other uses.
Styrofoam “clamshell” food containers, which are ubiquitous from take-out restaurants and shops in the cities and often wind up just floating away on ocean currents, never to be destroyed, are collected and brought to the biorefinery.
There they go into an acetone bath and the dissolved liquid blended with low-grade biochar and poured into molds to dry. The resulting hard resin is mold-proof, waterproof, non-degradable, lightweight and durable.
Depending on the dies and molds, it can become a whole range of products — roofing tile, caulk, surfboards, fishing boats, life-vests, doors, bicycles, and ice chests.
Image above: Detail of the network of uses of bamboo in a regenerative agroecosystem. Click for the whole enlarged image. From original article.
If there is a surge in demand for a particular product — refrigerator deodorizers or animal feed supplements, for instance — or there is a surplus of some particular feedstock — bamboo knocked down by a storm — the biorefinery can shift its production pattern to take advantage immediately.
This system sequesters more carbon than it emits, so we call it “cool.” By adding biochar, mineral rich compost, and microorganisms to the poor soils, we can jump-start soil productivity and boost farm productivity.
The gains in those alley-cropped contours will be anywhere from 40-percent to 400-percent vegetative growth, depending on the type of plants and the quality of the soils (poor soils will produce higher performance gains than good soils).
The same can be said for fish and livestock fed the leaf-protein and biochar nutriceuticals.
Let us pause here just a moment. Step back and take a look at the big picture. What is really being increased here is not so much village-scale well-being as photosynthesis. How are the greenhouse gases that are causing climate catastrophe — principally CO2, CH4 and N2O — to be removed from the atmosphere?
Mainly, although not exclusively, they will be removed by photosynthesis. The more of Earth’s surface that can be brought to bear on that task, the sooner the vital balance that harbors life on this tiny blue rock in space can be restored and the crisis ended.
Poultry can free-range the alleys to benefit of both plants and animals. Grazers can be moved through rotational cells that take advantage of water impoundments and high quality supergrasses. Fed nutrient-dense supplements with biochar, fish, poultry and grazing animals all grow faster and healthier without antibiotics or hormones, and deposit long-lived biochar back into the earth for long term carbon storage and soil fertility.
Growing nutrient-dense, no-till, organic food and perennial fibers on these marginal lands, using bioenergy and biofertilizers, creates a new, circular bioeconomy. There is no such thing as waste. Nothing need leave the system, but what does is not raw material or pollution — representing the depleting wealth of the land — but high value byproducts — providing return on social capital invested. Waste becomes an orphaned verb.
Transportation presents an energetic challenge in the post-petroleum world. Nearly all modern forms of transportation evolved in an era of cheap net energy and diminish in economic viability when costed on renewable sources and life cycles.
Gone will be diesel-powered semi-tractor-trailers and locomotives. There could be new generations of electrified tow-paths for barges and gondolas, mag-lev rail and other innovations, but these costly innovations will be fragile in an era marked by overpopulation, resource constraints, climate chaos and economic contraction and likely will not provide a stable foundation for commerce in most places. Returning will be sail and animal powered transport.
If taken to maximum scale (rotationally planting an area the size of India each year and installing Cool Labs in every village), at a capital cost of $10000 to $15000 per hectare, the price would tally up to approximately 2% of the price of the fairy dust BECCS (Biomass Energy with Carbon Capture and Storage) conversion favored by geoengineers stuck in the fossil industrial paradigm.
Moreover, while BECCS represents continuing cost and is fraught with risk from plantation biomass crops — possibly genetically engineered and carrying along the can of worms that opens up — hazardously supplanting forested, multi-diverse, self-regenerating ecosystems.
The Cool Lab alternative represents antifragile synergies of local conservation communities, continuous and adaptive profits, and continuous gains in ecological health, stability and wealth.
Can the conversion be done in time? In contrast to the 45-year gradual expansion of soybean cropping from the early 1960s to reach 200 Mha today, this system offers five times the protein per area farmed while providing a far greater, and more immediate, returns on investment.
When one considers the rapid growth of renewable energy in the past decade, consider this: an energy producing Cool Lab costs one-seventh the capital as hydro, wind or solar and runs entirely on “wastes” that would otherwise be destined to add greenhouse gases to the atmosphere but are now intercepted and neutralized.
Cool Labs use the existing financial and technological landscape of the world today and simply change the way products are produced in order to heal the earth, balance carbon, and make more real wealth for more people more quickly.
Does this hold a hazard in the form of perpetuating wealth inequality, militarism and hegemony by the “taker” class? Yes it does. However, in the post-petroleum era, relocalization of economies is inevitable, and with relocalization comes local control over shared destinies.
Cool Labs represent circular economies that are inherently leveling.
Each lab adapts to needs and available resources and can flex to provide more or less of a particular kind of benefit and tailor fuels to available feedstocks and labor options. The number of cascades possible is limited only by the imagination and each year we conceive of more. We are at the dawn of a new kind of lean, clean, nature-centered economy.
This system can turn almost any human settlement into an ecovillage, although the criteria for what defines ecovillage must necessary include a few more elements than merely having a Cool Lab or permacultural support systems.
Ecovillages are based on a cohesive worldview, an abiding respect for the ecological integrity of your home biome, a circular local economy and a culture of peace and mutual respect. Depending on your starting point for each of these elements, bringing all of them into harmony can take time and effort.
The energy and food production system using mixed-aged, mixed-species forest, wetland and marine ecosystems we’ve outlined, taken to scale on the world’s available marginal land (not productive farmland or developed areas) could restore the fertility of those soils and waters while sequestering carbon from the atmosphere at the average rate of 17 PgC/yr after getting established.
To get back to the Holocene we need to return atmospheric carbon to pre-industrial range, around 260 ppm. The system just described, at full scale, could do that within about 50 years, taking into account the oceans’ CO2 outgassing feedback.
Village scale Cool Labs could achieve the cumulative storage of 667 gigatons of legacy carbon required to bring atmospheric carbon back to pre-industrial levels in the lifetimes of the majority of people now living. Were nations to collectively phase out fossil fuels as quickly as called for in the Paris Agreement, restabilization of the climate would be achieved sooner.
Recovering one percentage point of soil organic matter means that around 27 long tons of organic matter per hectare would enter the soil and remain there. Because around two thirds of organic matter added to agricultural soils will be decomposed by soil organisms and plants and given back to the atmosphere, in order to add permanently 27 tons, a total of 81 tons of organic matter per hectare would be needed. This cannot be done quickly or it just washes or evaporates away. A slow process is required.
An example of how this could play out in Haiti or anywhere else can be seen in the Loess Plateau of Northern China where fertile soils were overworked until they had to be abandoned. At the time of abandonment organic carbon concentrations had dropped to under 3 percent.
Thirty years later Loess soils had regained concentrations of 6 percent by natural processes. If natural restoration were accelerated by amending soil carbon in both metabolizable forms (such as crop litter and manures) and recalcitrant forms (such as biochar), the potential to increase soil carbon in a few decades could be raised to 10 percent or greater. This could happen virtually anywhere.
A farm that switches to organic, animal powered no-tillage methods can sequester 1 to 4 tons of organic matter per acre per year. By employing perennial polycultures, rotated pastures of grazing animals, trees and wild plant strips, that amount can be doubled or tripled.
Harvard professor Thomas Goreau writes:
Carbon stored in the world’s soils and living biomass provides additional benefits beyond sequestration. As soil conditions improve, erosion and pests decline and the land comes back into balance.
Farming this way globally could sequester about 8 percent of the current total annual human-made emissions of 10 petagrams of carbon (PgC).
However, the fertility gains (equivalent to more than all of current global fertilizer production) would mean that chemical fertilizers could be (and should be) eliminated where carbon farming is practiced.
By reducing emissions of nitrous oxide from fertilizer (equivalent to approximately 8 percent annual human-made greenhouse gases) and the transportation and energy impacts of fertilizer production, we shave another 1 percent off global emissions.
But let’s keep going. If organic waste is returned to agricultural soils in the form of compost, then methane and CO2 emissions from its current destinations to landfills and wastewater (equivalent to 3.6 percent of man-made emissions) could be significantly reduced. Even a modest start, such as by elevating the soil carbon content of existing farmed soils by 0.4 percent, would have the potential to offset global greenhouse gas emissions by approximately 20 percent per year.
If biochar is added to the compost, we can quickly get to 100 percent, and then 120 percent. That is when it starts to matter.
After 10 years, we can increase progressively the reincorporation of organic matter into soils. By mid-21st century, we could increase the total world reservoir of carbon in the soil by two percentage points, and possibly more. In this way it is conceivable to restore our soil carbon reservoir to 10 percent, as Goreau argues. Because the system works best in poor soils, and because it eventually creates its own hydrological cycles, it can even re-green and reforest sandy deserts.
Are we doomed to Near Term Human Extinction?
Not yet. While there are still wild cards waiting to be played, what we have outlined shows a complete escape from our present trajectory. Is it possible that technology no more complicated than an Easy Bake Oven — and that pays for itself — can reverse climate change?
Image above: Photo of Chinese model of a Cool Lab rotary oven. From original article.
The rotary oven pictured above gasifies waste rice husks at the rate of 2.5 tons per hour. Thirty-five percent of that weight is transformed into biochar.
Half of the rest, as pyrogas, is extracted for useful synthetic compounds that replace petrochemicals.
The other half of that gas is used to co-generate 1.6 megawatts of electricity from this half-million-dollar biorefinery. It could also be refined into a liquid substitute for gasoline.
The Chinese government has invested heavily to develop this technology, and the wares they are producing are now the most efficient and lowest cost in the world. They will pour another $40 million into advanced biochar research this year.
Chinese Cool Lab reactors have been sold to 20 countries, including Haiti. In Senegal there is a prototype that has been continuously operating for 8 years.
In Egypt, the biochar made by their Chinese reactor is producing organic cabbages from the sandy shore of the Suez Canal. We witnessed a similar effect in the infertile clay soil beside the Asian Biochar Centre in Nanjing.
This we know: we can achieve faster and more well-rounded human development within the carrying capacity of the Earth. Will we? Who decides?
.
By Albert Bates on 2 April 2017 for The Great Change -
(http://peaksurfer.blogspot.co.uk/2017/04/the-cool-lab.html)

Image above: Illustration of "biorefinery" concept that can be applied to utilization of bamboo forestry. From original article.
[IB Publisher's note: The "Cool Lab" technology that Albert Bates describes in this article includes ideas from holistic thinking, permaculture, and technologies like biochar that could transform agriculture and our use of technology, and be the solution to Global Warming, Climate Change and our own extinction. Get on board!]
In Permaculture the first stage of any design is protracted observation. What does a biological system have in over-abundance? What is scarce? How will it restore balance? What are the obstacles?
Let us say that an impoverished village in Haiti risks being carried away by mudslides that follow brush fires where the forest has been cut down to supply wood for shelter and cooking.
What things are scarce? In no particular order:
- food
- water
- cooking fuel
- secure shelter
- energy
- productive employment
- biodiversity soil
- birth control
- health care
- mud
- deforestation
- rain
- hurricanes
- earthquakes
- unemployed people
- superstition
- resentment
- mosquitoes
- climate change
The hillside needs to be planted with vegetation. It is especially important that the hilltops be forested.
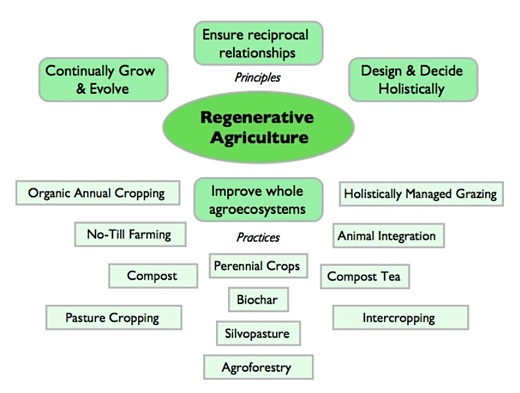
Image above:Principles and techniques Refenerative Agriculture to improve whole agroecosystems. From original article.
A keyline analysis will show us where water wants to go when it rains, and how best it can be held high in the landscape and directed both to subsurface flows and to dam storage for uses in the dry season. Alley cropping along the contours follows hand-cut swales (or machine cut where financial capital substitutes for social capital).
The berms are planted with successional understory (in this tropical example, pineapple, cassava, ginger, allspice, coffee and medicinal herbs), mid-level canopy banana, papaya, moringa, cacao, mulberry, tree legumes of mimosa, cassia, and pea subfamilies, chaya, climbing vines such as vanilla, dioscorea, cucumber, chocho and pasaflora, and eventual overstory of coconut, jackfruit, breadfruit, breadnut, ramon, samwood, mahogany, cedar, bamboo, peach palm, etc.
Between the alleys are seeded perennials such as callalu, okra, sorghum, and supergrasses like kernza (Thinopyrum intermedium), sunn hemp (Crotalaria juncea), pennisitum and pearl millet hybrids (Tembo), brassica napus, amaranth, etc., as well as familiar food crops such as maize, rice, yam and beans, where soils and water supply are well suited.
As much as possible, the planting process can be accompanied by biofertilizers having a high percentage of finely pulverized biochar, activated indigenous microorganisms, some immediate food for those microbes (such as composted food wastes and manures), and minerals keyed to redress local soil deficiencies.
If these biofertilizers are not immediately available for the first plantings, they can always be added later, as a byproduct of the early harvests.
Water in storage on the hillsides is edge-planted with Acoris, a plant that inoculates the water with a mosquito-larvae destroying resin. As the Acoris matures, pools and dams progress from being mosquito generating to mosquito decimating.
In the lowlands, water that overflows from catchments above is directed into chinampas, constructed wetlands composed of alternating islands and channels and rotating between aerobic (horizontal and vertical flow reedbeds) and anaerobic (settling lagoons) seeded with aquatic and semi-aquatic plants (taro, Chinese water spinach, lotus, azola, wild rice) and freshwater fish (aquaculture).
Acoris for mosquito control can also be planted here, but the fish do most of that work already, so the plant is only needed in mudflats and places fish cannot go.
The appearance of this microbiome also augurs the reappearance of frogs, peepers, lizards, dragonflies, water birds, bats, turtles, and forest mammals who venture to the water’s edge to drink.
Within the first season, the hillside mud problem is erased, deforestation is reversed, and food scarcity begins to be alleviated from the fast-yielding varieties of annuals, perennials and fish.
Productive employment can expand this system as much as available land permits, even on relatively steep hillsides. Resentment diminishes, and with it, superstition.
Within the village a regenerative, biological energy system arrives to replace the fossil fuel (diesel electric) grid-based source that previously had supplied electricity only intermittently, occasionally dimming lights and frying phone chargers and boom boxes.
This system consists of a biomass furnace, running on the woody wastes from coppice (the moringa, jackfruit and cassava plantation), coconut, rice or other shell crops, pelletized supergrasses and other biomass after food harvest or extraction of leaf protein, vitamins and useful fiber.
The loading dock at the biorefinery receives raw materials second-harvested from the farms.
Leaves of tropical legumes (Leucaena Zeucocephala, Vigna unguiculata, Clitoria ternatea, Desmodium distortum, Psophocarpus tetragonolobus, Macroptilium lathyroides, Phaseolus calcaratus, Brassica napus, and Manihot esculenta, for instance) are taken by conveyor and chopped into 2-cm pieces, soaked in 2-percent sodium metabisulfite, disintegrated in a hammer mill and pressed in a single-screw press.
The expressed juice is heated with steam (produced by the furnace) and protein coagulum collected, centrifuged, and pressed, then spread in a thin layer on glass plates and dried in an air-filtered, dehumidified room. It is then collected as a powder and containerized to be used or sold as a feed supplement.
At its most basic level, high-protein, high-quality leaf protein fractionation is simple. Production is geared to consumption by farm animals to remove some of the food safety, preservation and storage concerns.
Later improvements can produce dried leaf extracts for human consumption but higher capital costs are incurred and clean-room protocols by workers become essential.
Following leaf-protein extraction, the dried mash from the press is used as a feedstock for the furnace, where it joins other dried agricultural wastes: coppice wood, prunings, bamboo thinnings, pallets, cardboard boxes, coconut coir, nut and rice husks, etc.
All of this is pyrolyzed, the heat captured to run both the leaf protein process and produce electricity, and co-products (fractionated volatile gases, wood vinegar) drawn off before the final product — high quality biochar — remains.
The biochar is quenched (preferably with urine because that adds a 30% fertility gain), pulverized, and charged (blended with microbe-rich aerobic compost) to make a potent “cool” biofertilizer.
Alternatively, it is kept at food-grade and sold as a dry product for use as a food supplement, animal feed probiotic, water filtration medium or deodorizer. At less-than-food-grade it can be used as a litter amendment to reduce smells in animal enclosures, improve the fermentation of silage, or go into a variety of natural building materials — paints, dyes, plasters, wallboard and bricks.
And it can always become biofertilizer, even after undergoing one or more of these other uses.
Styrofoam “clamshell” food containers, which are ubiquitous from take-out restaurants and shops in the cities and often wind up just floating away on ocean currents, never to be destroyed, are collected and brought to the biorefinery.
There they go into an acetone bath and the dissolved liquid blended with low-grade biochar and poured into molds to dry. The resulting hard resin is mold-proof, waterproof, non-degradable, lightweight and durable.
Depending on the dies and molds, it can become a whole range of products — roofing tile, caulk, surfboards, fishing boats, life-vests, doors, bicycles, and ice chests.

Image above: Detail of the network of uses of bamboo in a regenerative agroecosystem. Click for the whole enlarged image. From original article.
This system sequesters more carbon than it emits, so we call it “cool.” By adding biochar, mineral rich compost, and microorganisms to the poor soils, we can jump-start soil productivity and boost farm productivity.
The gains in those alley-cropped contours will be anywhere from 40-percent to 400-percent vegetative growth, depending on the type of plants and the quality of the soils (poor soils will produce higher performance gains than good soils).
The same can be said for fish and livestock fed the leaf-protein and biochar nutriceuticals.
Let us pause here just a moment. Step back and take a look at the big picture. What is really being increased here is not so much village-scale well-being as photosynthesis. How are the greenhouse gases that are causing climate catastrophe — principally CO2, CH4 and N2O — to be removed from the atmosphere?
Mainly, although not exclusively, they will be removed by photosynthesis. The more of Earth’s surface that can be brought to bear on that task, the sooner the vital balance that harbors life on this tiny blue rock in space can be restored and the crisis ended.
Poultry can free-range the alleys to benefit of both plants and animals. Grazers can be moved through rotational cells that take advantage of water impoundments and high quality supergrasses. Fed nutrient-dense supplements with biochar, fish, poultry and grazing animals all grow faster and healthier without antibiotics or hormones, and deposit long-lived biochar back into the earth for long term carbon storage and soil fertility.
Growing nutrient-dense, no-till, organic food and perennial fibers on these marginal lands, using bioenergy and biofertilizers, creates a new, circular bioeconomy. There is no such thing as waste. Nothing need leave the system, but what does is not raw material or pollution — representing the depleting wealth of the land — but high value byproducts — providing return on social capital invested. Waste becomes an orphaned verb.
Transportation presents an energetic challenge in the post-petroleum world. Nearly all modern forms of transportation evolved in an era of cheap net energy and diminish in economic viability when costed on renewable sources and life cycles.
Gone will be diesel-powered semi-tractor-trailers and locomotives. There could be new generations of electrified tow-paths for barges and gondolas, mag-lev rail and other innovations, but these costly innovations will be fragile in an era marked by overpopulation, resource constraints, climate chaos and economic contraction and likely will not provide a stable foundation for commerce in most places. Returning will be sail and animal powered transport.
If taken to maximum scale (rotationally planting an area the size of India each year and installing Cool Labs in every village), at a capital cost of $10000 to $15000 per hectare, the price would tally up to approximately 2% of the price of the fairy dust BECCS (Biomass Energy with Carbon Capture and Storage) conversion favored by geoengineers stuck in the fossil industrial paradigm.
Moreover, while BECCS represents continuing cost and is fraught with risk from plantation biomass crops — possibly genetically engineered and carrying along the can of worms that opens up — hazardously supplanting forested, multi-diverse, self-regenerating ecosystems.
The Cool Lab alternative represents antifragile synergies of local conservation communities, continuous and adaptive profits, and continuous gains in ecological health, stability and wealth.
Can the conversion be done in time? In contrast to the 45-year gradual expansion of soybean cropping from the early 1960s to reach 200 Mha today, this system offers five times the protein per area farmed while providing a far greater, and more immediate, returns on investment.
When one considers the rapid growth of renewable energy in the past decade, consider this: an energy producing Cool Lab costs one-seventh the capital as hydro, wind or solar and runs entirely on “wastes” that would otherwise be destined to add greenhouse gases to the atmosphere but are now intercepted and neutralized.
Cool Labs use the existing financial and technological landscape of the world today and simply change the way products are produced in order to heal the earth, balance carbon, and make more real wealth for more people more quickly.
Does this hold a hazard in the form of perpetuating wealth inequality, militarism and hegemony by the “taker” class? Yes it does. However, in the post-petroleum era, relocalization of economies is inevitable, and with relocalization comes local control over shared destinies.
Cool Labs represent circular economies that are inherently leveling.
Each lab adapts to needs and available resources and can flex to provide more or less of a particular kind of benefit and tailor fuels to available feedstocks and labor options. The number of cascades possible is limited only by the imagination and each year we conceive of more. We are at the dawn of a new kind of lean, clean, nature-centered economy.
This system can turn almost any human settlement into an ecovillage, although the criteria for what defines ecovillage must necessary include a few more elements than merely having a Cool Lab or permacultural support systems.
Ecovillages are based on a cohesive worldview, an abiding respect for the ecological integrity of your home biome, a circular local economy and a culture of peace and mutual respect. Depending on your starting point for each of these elements, bringing all of them into harmony can take time and effort.
The energy and food production system using mixed-aged, mixed-species forest, wetland and marine ecosystems we’ve outlined, taken to scale on the world’s available marginal land (not productive farmland or developed areas) could restore the fertility of those soils and waters while sequestering carbon from the atmosphere at the average rate of 17 PgC/yr after getting established.
To get back to the Holocene we need to return atmospheric carbon to pre-industrial range, around 260 ppm. The system just described, at full scale, could do that within about 50 years, taking into account the oceans’ CO2 outgassing feedback.
Village scale Cool Labs could achieve the cumulative storage of 667 gigatons of legacy carbon required to bring atmospheric carbon back to pre-industrial levels in the lifetimes of the majority of people now living. Were nations to collectively phase out fossil fuels as quickly as called for in the Paris Agreement, restabilization of the climate would be achieved sooner.
Recovering one percentage point of soil organic matter means that around 27 long tons of organic matter per hectare would enter the soil and remain there. Because around two thirds of organic matter added to agricultural soils will be decomposed by soil organisms and plants and given back to the atmosphere, in order to add permanently 27 tons, a total of 81 tons of organic matter per hectare would be needed. This cannot be done quickly or it just washes or evaporates away. A slow process is required.
An example of how this could play out in Haiti or anywhere else can be seen in the Loess Plateau of Northern China where fertile soils were overworked until they had to be abandoned. At the time of abandonment organic carbon concentrations had dropped to under 3 percent.
Thirty years later Loess soils had regained concentrations of 6 percent by natural processes. If natural restoration were accelerated by amending soil carbon in both metabolizable forms (such as crop litter and manures) and recalcitrant forms (such as biochar), the potential to increase soil carbon in a few decades could be raised to 10 percent or greater. This could happen virtually anywhere.
A farm that switches to organic, animal powered no-tillage methods can sequester 1 to 4 tons of organic matter per acre per year. By employing perennial polycultures, rotated pastures of grazing animals, trees and wild plant strips, that amount can be doubled or tripled.
Harvard professor Thomas Goreau writes:
Current rates of carbon farming at typical current levels would take thousands of years to draw down the dangerous excess CO2, but state of the art methods of soil carbon sequestration could draw it down in as little as decades if the percentage of long lived carbon is raised to as little as about 10%.If the recuperation of soil carbon became a central goal of agricultural policies worldwide, it would be possible and reasonable to set as an initial goal the sequestration of one half ton per acre-year (1.5 t/ha-y or 500 grams per m2/y), comparable to the 4 pour 1000 program (4 grams per kg of soil) proposed by the French delegation at COP-21.
Carbon stored in the world’s soils and living biomass provides additional benefits beyond sequestration. As soil conditions improve, erosion and pests decline and the land comes back into balance.
Farming this way globally could sequester about 8 percent of the current total annual human-made emissions of 10 petagrams of carbon (PgC).
However, the fertility gains (equivalent to more than all of current global fertilizer production) would mean that chemical fertilizers could be (and should be) eliminated where carbon farming is practiced.
By reducing emissions of nitrous oxide from fertilizer (equivalent to approximately 8 percent annual human-made greenhouse gases) and the transportation and energy impacts of fertilizer production, we shave another 1 percent off global emissions.
But let’s keep going. If organic waste is returned to agricultural soils in the form of compost, then methane and CO2 emissions from its current destinations to landfills and wastewater (equivalent to 3.6 percent of man-made emissions) could be significantly reduced. Even a modest start, such as by elevating the soil carbon content of existing farmed soils by 0.4 percent, would have the potential to offset global greenhouse gas emissions by approximately 20 percent per year.
If biochar is added to the compost, we can quickly get to 100 percent, and then 120 percent. That is when it starts to matter.
After 10 years, we can increase progressively the reincorporation of organic matter into soils. By mid-21st century, we could increase the total world reservoir of carbon in the soil by two percentage points, and possibly more. In this way it is conceivable to restore our soil carbon reservoir to 10 percent, as Goreau argues. Because the system works best in poor soils, and because it eventually creates its own hydrological cycles, it can even re-green and reforest sandy deserts.
Are we doomed to Near Term Human Extinction?
Not yet. While there are still wild cards waiting to be played, what we have outlined shows a complete escape from our present trajectory. Is it possible that technology no more complicated than an Easy Bake Oven — and that pays for itself — can reverse climate change?

Image above: Photo of Chinese model of a Cool Lab rotary oven. From original article.
The rotary oven pictured above gasifies waste rice husks at the rate of 2.5 tons per hour. Thirty-five percent of that weight is transformed into biochar.
Half of the rest, as pyrogas, is extracted for useful synthetic compounds that replace petrochemicals.
The other half of that gas is used to co-generate 1.6 megawatts of electricity from this half-million-dollar biorefinery. It could also be refined into a liquid substitute for gasoline.
The Chinese government has invested heavily to develop this technology, and the wares they are producing are now the most efficient and lowest cost in the world. They will pour another $40 million into advanced biochar research this year.
Chinese Cool Lab reactors have been sold to 20 countries, including Haiti. In Senegal there is a prototype that has been continuously operating for 8 years.
In Egypt, the biochar made by their Chinese reactor is producing organic cabbages from the sandy shore of the Suez Canal. We witnessed a similar effect in the infertile clay soil beside the Asian Biochar Centre in Nanjing.
This we know: we can achieve faster and more well-rounded human development within the carrying capacity of the Earth. Will we? Who decides?
.
No comments :
Post a Comment